ABSTRACT
From October 2020 to September 2021, in two forest microcatchments in the Czech Republic, the quality of wet atmospheric deposition (bulk and throughfall) was monitored simultaneously with the surface water quality in the local watercourse, humus, and the moss species Pleurozium schreberi. An evaluation is presented of the 15 polycyclic aromatic hydrocarbons (PAHs) burden of the above-mentioned matrices. The first site was chosen in the Beskid Mountains in the Moravian-Silesian region, in the cadastre of the village of Bystřice in the upper basin of the Suchý stream (altitude 590 to 835 m a.s.l.). This area is affected by industrial activities. The second reference site was chosen in the Bohemian-Moravian Highlands near Košetice observatory (altitude 520 m a.s.l.). A significant PAHs burden was confirmed at the Bystřice site. The concentration of Σ15 PAH during the monitored period in the bulk deposition was 0.785 ± 0.579 mg.l-1 at the Bystřice site and 0.114 ± 0.110 mg.l-1 at the Košetice site. In throughfall deposition, the concentration of Σ15 PAHs was slightly higher: 0.824 ± 0.670 mg.l-1 in Bystřice and 0.203 ± 0.141 mg.l-1 in Košetice. Significantly higher PAHs concentrations were found in the cold half of the year. The amount of atmospheric deposition of Σ15 PAHs in Bystřice was calculated at 1,098.7 g.km-2.year-1; in Košetice it is 10 times lower at 102.7 g.km-2.year-1. The topsoil and vegetation cover PAHs sorb. PAHs enter surface waters through erosion. The concentration of Σ15 PAHs in the Suchý stream at the Bystřice site was 0.026 ± 0.049 mg.l-1, while in the Lesní stream at the Košetice site it was 0.033 ± 0.038 mg.l-1. Total Σ15 PAHs flux by the Suchý stream (upper basin) accounts for only 1 % of the atmospheric bulk deposition in Bystřice and 2.8 % by the Lesní stream in Košetice. The ratio of fluoranthene and pyrene in the precipitation indicates the origin of PAHs pollution from combustion processes (FLT/PYR > 1) at both sites. In bulk deposition, this FLT/PYR ratio was 1.6 in Bystřice and 1.5 in Košetice, and 1.5 (Bystřice) and 1.6 (Košetice) in the throughfall. The river sediment burden with Σ15 PAHs in the Lesní stream (1.498 ± 0.138 mg.kg-1) was more than in the Suchý stream (0.340 ± 0.109 mg.kg-1) due to the different granularity with a significantly higher proportion of fine soil particles, although the content of Σ15 PAHs in the upper soil layer was 3.2 to 3.7 times lower in Košetice than in Bystřice. Similarly, the content of Σ15 PAHs in Pleurozium schreberi was 3 times lower at Košetice than at the exposed Bystřice site.
INTRODUCTION
Polycyclic aromatic hydrocarbons (PAHs) form an important group of substances, most of which show adverse effects on aquatic organisms and humans. Due to their persistence, they have the ability to remain in the aquatic environment for a long time. Directive 2008/105/EC of the European Parliament and of the Council [1], as amended by Directive 2013/39/EU [2], included selected PAH substances in the list of priority substances, of which anthracene, benzo[a]pyrene, benzo[b]fluoranthene, benzo[k]fluoranthene, benzo[g,h,i]perylene, and indeno[1,2,3-cd]pyrene are identified as priority hazardous substances. According to Framework Directive
2000/60/EC establishing the framework for Community action in the field of water policy [3], Article 16 (Strategy against pollution of water) there is a need to reduce discharges, emissions, and leaks of these substances in a targeted manner; in the case of priority dangerous substances it is even a matter of stopping or gradual elimination of input into the environment. The requirements of the above Directives were implemented in Czech Government Radulation No. 401/2015 Coll. [4].
Polycyclic aromatic hydrocarbons are ubiquitous substances found in all components of the environment. They are one of the most common reasons for failure to achieve good chemical and ecological status of surface waters (note: PAHs specified as priority substances are currently subject to chemical status assessment, other PAHs belong to the group of specific pollutants, which are one of the components of the ecological status assessment of surface water bodies). The environmental quality standard expressed as an annual average is the strictest for benzo[a]pyrene (0.17 ng.l-1), followed by fluoranthene (6.3 ng.l-1) [4].
This was also the reason for the inclusion of the group of PAH substances in the TA CR project SS01010231 “Impacts of atmospheric deposition on the aquatic environment with consideration of climatic conditions”, which was realized from March 2020 to December 2022. The aim of this project was to verify the level of pollution in selected components of the environment, or to investigate the link between them with the impact on surface water quality in order to be able to better quantify this impact in the future, and to propose effective measures for achieving a good chemical status of surface waters in terms of PAH pollution. Two different forest microcatchment sites were chosen: one with significant anthropogenic influence (the upper part of the Suchý stream basin in the cadastre of Bystřice municipality in the Moravian-Silesian Beskids) and the other in the reference area (the Lesní stream in the cadastre of Košetice municipality near Košetice climatological station).
POLYCYCLIC AROMATIC HYDROCARBONS IN THE ENVIRONMENT
Polycyclic aromatic hydrocarbons (PAHs) can be found in all components of the environment. This is due to the fact that the dominant source of pollution is combustion processes, which are of both natural and anthropogenic origin. The most important natural sources of PAHs are volcanic activity, vegetation cover fires, and some sedimentary rocks. Anthropogenic emissions of PAHs of a non-industrial nature arise from the targeted burning of vegetation, from domestic heating, and smoking. In industry, the dominant sources of pollution are the production of coke, electric and thermal energy, smelters, selected branches of chemical industry (tar processing, catalytic cracking, soot production), and also food industry [5].
The rate of PAH production depends on the combustion process and the type of fuel used. It is highest during incomplete combustion, which happens mostly in local domestic heating. The mechanism of PAH formation involves two processes: pyrolysis and pyrosynthesis. Pyrolysis produces PAH precursors, which recombine at temperatures of 500 to 800 °C to form relatively stable aromatic hydrocarbons [5]. In the case of incomplete combustion, the emissions of primary PAHs contained in the fuel can also occur. The primary emissions of PAHs into the air are predominantly in the gaseous phase, but their condensation and sorption to fine dust particles occurs relatively quickly during the cooling of flue gases. The rate of sorption depends on the molecular weight. According to selected characteristics of physico-chemical properties (Henry’s constant, partition coefficients Kow, Koc) we can divide PAHs into:
- low molecular weight (152 to 178 g.mol-1) – acenaphthene, acenaphthylene, anthracene, phenanthrene and fluorene (consisting of 2 to 3 aromatic nuclei),
- medium molecular weight (202 g.mol-1) – fluoranthene, pyrene (consisting of 4 aromatic nuclei),
- high molecular weight (228 to 278 g.mol-1) – benzo[a]anthacene, benzo[b]fluoranthene, benzo[k]fluoranthene, benzo[a]pyrene, benzo[g,h,i]perylene, dibenzo[a,h]anthracene, chrysene, indeno[1,2,3-c,d]pyrene (consisting of 5 or more aromatic nuclei) [6].
This division is important because the above-mentioned groups of PAHs behave differently in the environment. We can show the differences, for example, using Henry’s constant, which states the partial pressure of the gas above the solution, expressed in the unit Pa.m3.mol-1. For naphthalene it is 43.00, acenaphthene 12.17, pyrene 0.919, and benzo[k]fluoranthene 0.044 Pa.m3.mol-1 [7]. The difference is within several orders of magnitude; the higher the molecular weight, the easier and faster the binding to fine particles.
When burning coal, mainly phenanthrene (over 50 %), anthracene, and fluoranthene to a lesser extent, and a small amount of benzo[a]pyrene (0.5 to 2.4 %) are produced [6]. Combustion products are also PAH derivatives, mainly nitroaromatics.
In the atmosphere, mainly low molecular weight PAHs are broken down by sunlight. High molecular weight PAHs are sorbed to particles of different sizes. The smaller the particles, the longer the degradation time is needed to break down the PAH (up to several weeks), and the longer the PAHs remain in the atmosphere. From the atmosphere, PAHs are introduced into other components of the environment by dry and wet deposition. Due to their longer lifetime, high-molecular-weight PAHs are transported from the source over long distances, depending on climatic conditions and the time of year. In winter, the concentration of PAH in the air is significantly higher than in summer. This is due to higher emissions from combustion processes combined with lower efficiency of photodegradation processes in the cold part of the year.
From the atmosphere, PAHs reach vegetation and the earth’s surface through dry and wet deposition. On agricultural soils, PAHs penetrate into deeper soil layers due to ploughing, while in other cases they remain in surface layers. Low molecular weight PAHs partially volatilize back into the atmosphere or are decomposed by photochemical processes. Biodegradation by microorganisms is also present, which is the predominant factor in the decomposition of primary PAHs. The rate of degradation depends on the soil type and organic carbon content. S. Thiele-Bruhn studied the kinetics of PAH degradation in soils contaminated by industrial activity (gas industry, coke plants) [8]. Fine soil with a particle size below 2 mm from 11 sites with a predominance of loamy-sandy soils was placed in Mitscherlich containers and fertilized with equal amounts of phosphorus and potassium in order to stimulate microbial processes. The experiment took place for 168 weeks in natural conditions. The result was the determination of the degradation rate constant “k” and the decrease of individual PAHs expressed as DT50 (disappearance time). In the case of naphthalene and acenaphthene, the median DT50 was units of weeks (6.1 and 9.5, respectively), for anthracene and phenanthrene tens of weeks (70 and 92, respectively), for other high molecular weight PAHs it was over 100 weeks with a maximum of 522 weeks for benzo[k]fluoranthene. Thus, high molecular weight PAHs remain in the soil for a long time.
Particularly low molecular weight PAHs with 2 to 3 aromatic nuclei, which make up to 80 % of total PAHs, pass into the vegetation from the soil and the atmosphere through the root system and leaves. The relatively high concentration of naphthalene in crops is due to its higher solubility in water [9]. High molecular weight PAHs are sorbed on the surface of vegetation. PAHs reach surface waters through erosional washes from soils, vegetation, and from the paved surfaces of roads and urban agglomerations. This type of transfer in terrestrial systems dominates over bulk deposition. High molecular weight PAHs preferentially bind to fine particles of undissolved substances in water, and sediment in suitable places depending on the nature of the flow. In well-oxygenated streams, the process of PAH degradation is faster, both in the water column and in river sediment. The rate of microbial revival of the aquatic environment also plays a positive role in the process of their degradation. The present dissolved organic matter (DOM) accelerates the photodegradation of low molecular weight PAHs by facilitating the formation of reactive intermediates and, conversely, inhibits the photodegradation of high molecular weight PAHs (e.g., benzo[a]pyrene) by binding their molecules [10].
In surface waters, PAHs are a long-term cause of failure to achieve good chemical status. In the last evaluated three-year period from 2016 to 2018, a total of 54.7 % of surface water bodies failed or were not classified in the fluoranthene indicator and 99.3 % in the benzo[a]pyrene indicator [11]. The latter indicator is also problematic from the point of view of the difficulty of achieving a sufficiently low limit of determination by laboratory techniques in relation to the value of the environmental quality standard (EQS) expressed as an annual average.
The ubiquity of PAHs in the environment, the failure to achieve good water quality, and the danger to human health are the reasons why it is necessary to pay attention to these substances. The International Agency for Research on Cancer (IARC) has classified 60 polycyclic aromatic hydrocarbons into groups according to their potential carcinogenic effects to humans. Of the 15 PAHs monitored as part of the ATMDEP project, benzo[a]pyrene belongs to group 1 – “proven carcinogen”. Group 2A – “probably carcinogenic to humans” includes dibenzo[a,h]anthracene. Group 2B – “suspected human carcinogen” includes benzo[a]anthracene, benzo[b]fluoranthene, benzo[k]fluoranthene, chrysene, indeno[1,2,3-c,d]pyrene. Group 3 – “unclassifiable” includes acenaphthene, anthracene, benzo[g,h,i]perylene, fluoranthene, fluorene, phenanthrene and pyrene. Benzo[a]pyrene is currently the only PAH representative in Group 1. In the human body, it metabolizes to PaP-7,8-diol 9,10-epoxide, which can damage DNA. For Group 3, there is still insufficient evidence of their carcinogenic effects [12]. It has to be noted that PAHs act in the mixture. Therefore, a number of authors have developed toxic equivalent factors (TEFs) for individual PAHs, which are related to the toxicity of benzo[a]pyrene (BAP = 1). Nisbet and LaGoy did so in 1992 [13]. They applied a higher TEF than for BAP in the case of DBA (TEF = 5). In the case of the other four PAHs (BAA, BBF, BKF, INP), TEF = 0.1. For ANT, BGP, and CHRY they applied TEF = 0.01. For other PAHs, the TEF is equal to 0.001. (The abbreviations used to denote PAHs are listed in Tab. 2.) Multiplying the concentration of each determined PAH by this factor and adding them up gives the equivalent concentration with respect to the toxic potential of benzo[a]pyrene.
METHODOLOGY
In the project, PAHs were investigated and evaluated which cause a failure to achieve good water status and, at the same time, which are expected to be transmitted through the air even at great distances from the sources of pollution.
In order to compare the presence of polycyclic aromatic hydrocarbons in individual components of the environment, samples of the following matrices were selected at the two sites:
- bulk, (monthly precipitation*),
- throughfall, (monthly rainfall*),
- surface water (monthly),
- river sediment (twice during the year),
- humus – a biologically stable humification layer (H, Oh horizon), after removal of forest fallout (Ol) and fermentation horizon (Of) (2x during the year – samples represent accumulated PAH deposition over a longer period of time – age of the forest),
- red-stemmed feathermoss (Pleurozium schreberi) (twice during the year – samples represent the average PAH deposition over the last three years of moss growth).
* For the analytical determination of polycyclic aromatic hydrocarbons in atmospheric deposition, it was necessary to obtain a sufficient volume of samples. At the Košetice site, there were three cases of insufficient amount of sample for analytical determination due to the low monthly rainfall total; therefore, in these cases, the rainfall samples were taken after a two-month exposure.
In aqueous samples, PAHs were analysed on an Agilent 1260 Infinity II liquid chromatograph with fluorescence detection. A Pinnacle II PAH 4 µm column, 150 x 4.6 mm (Restek), and a mobile phase with the composition A: methanol, B: water + 5 % methanol were used for separation. Sediments were lyophilized and sieved through a 2 mm sieve prior to extraction.
Polycyclic aromatic hydrocarbons in moss and humus samples were analysed on a Bruker EVOQ GC-TQ gas chromatograph by MS/MS. Red-stemmed feathermoss samples were collected in the autumn of 2020 and 2021 at three sites in the upper parts of the Suchý stream basin and in the vicinity of the Lesní stream in bulk (unaffected by throughfall deposition) in aluminium bags. After being transported to the laboratory in a cooling box, the moss samples were stored in a freezer and, after thawing, manually cleaned of unwanted impurities. For PAH determination, the upper green parts of the moss were torn off. The moss was then homogenized in a vibrating mill using liquid nitrogen and dried by lyophilization. PAHs were extracted with n-hexane. After evaporation, the extract was purified by gel permeation chromatography. Bio-Beads SX-3 styrenedivinylbenzene polymer gel was used. Humus samples were simultaneously collected in aluminium bags from the visually undisturbed Oh horizon at three sites in each micro-catchment and transported and stored like the moss samples. After drying by lyophilization, they were sieved to a size of 0.25 mm. PAHs were extracted with dichloromethane with the addition of Al2O3 and diatomaceous earth. PAH extractions from both moss and humus samples were performed at elevated temperature and pressure with a Dionex ASE 350 extractor.
For the project, model forest micro-catchments were selected that met the following criteria:
- proximity to CHMI monitoring points to monitor the amount of precipitation,
- sufficient number of places with red-stemmed feathermoss (Pleurozium schreberi),
- detection of anthropogenic influence on selected sites,
- sufficient water level throughout the sampling period (even in the case of low flows in the summer),
- minimizing the risk of theft or damage to rain gauges by strangers,
- suitability of the sites in terms of prevailing wind direction and landscape relief,
- integrated micro-catchment for monitoring the quality of atmospheric precipitation, surface water, and other environmental matrices.
Fig. 1. Location of the selected pilot sites
The following micro-catchments were selected as pilot areas on the basis of the above-mentioned criteria:
- The model basin of the Suchý stream is located in the Moravian-Silesian Beskids east of the Ostrava and Třinec agglomerations (between Třinec and Jablunkov), which, due to the prevailing air flow, is heavily loaded by exposures to polycyclic aromatic hydrocarbons from the local metallurgical and power engineering industries. In Jablunkovská brázda, local heating plants from concentrated and scattered buildings are also an important source of emissions. The share of long-distance transmission in the total load of PAHs in the Třinec area, characterized by suspended PM2.5 particles, is up to 10 % [14]. In its upper part, the Suchý stream valley is closed from the south by Javorový hill (627 m a.s.l.), which, towards the east, creates a ridge connected to the main ridge formed by the peaks of Polední (672 m a.s.l.) – Hrbel (727 m a.s.l.) – Loučka (835 m a.s.l.) and Filipka (771 m a.s.l.). The Suchý stream valley is open to the west towards the Třinec and Bystřice agglomeration. About 70 % of the upper part of the Suchý stream basin is made up of forests, the rest is meadows. Mixed forests prevail, with spruce in the highest parts. Beech stands make up to 85 % in and above the selected site. There is no direct source of pollution in the original upper part of the Suchý stream model area. The area of the model part of the basin is 0.462 km2. The Suchý stream is part of the basin of the HOD_750 – Hluchová water body from the spring to the confluence with the Olše, which, in the third planning cycle, does not reach good status due to higher PAH concentrations. In the text, the Suchý stream basin (Fig. 2) is referred to as BY after the name of the nearest village, Bystřice.
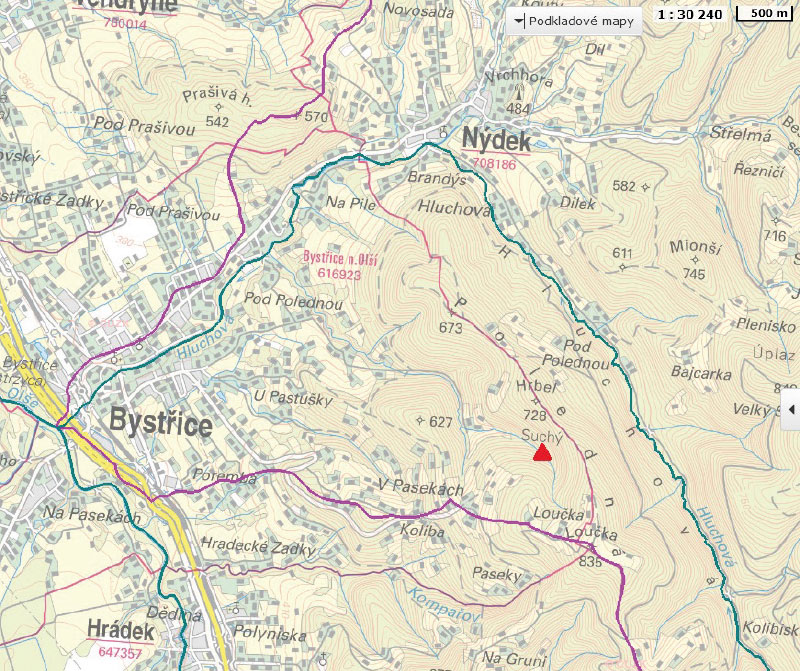
Fig. 2. Suchý stream site, Bystřice (Source: HEIS TGM WRI)
- The Lesní stream model basin, located in the Bohemian-Moravian Highlands northwest of the village of Košetice in the Borek forest near the middle part of the Anenský stream, river km 0.7, which then flows into the Martinický stream at 23.1 river km. The Lesní stream basin is part of the long-term integrated monitoring of environmental components of the Košetice National Atmospheric Observatory. The monitored basin is located 1 km south of the observatory; its area is 0.292 km2. Approximately 90 % of the basin is forested, the rest is agricultural land. The forested part is mostly covered with spruce monocultures; the predominant stands are around 90 years old with a mixture of pine, beech, larch, and birch. The Lesní stream is the only permanent tributary of the Anenský stream. The stream is part of the DVL_0440 Martinický stream basin, which achieved good chemical status in the second and third planning cycles, and environmental quality standards according to Government Regulation No. 401/2015 Coll., were not exceeded in terms of PAH indicators. The site is not in an area with a significant PAH deposition; it is outside continuous settlements and outside the direct reach of significant sources of pollution. Therefore, it was chosen as a suitable reference site for comparison with the selected more anthropogenically loaded site of Bystřice. In the text, the Lesní stream basin (Fig. 3) is designated KO after the nearest village of Košetice.
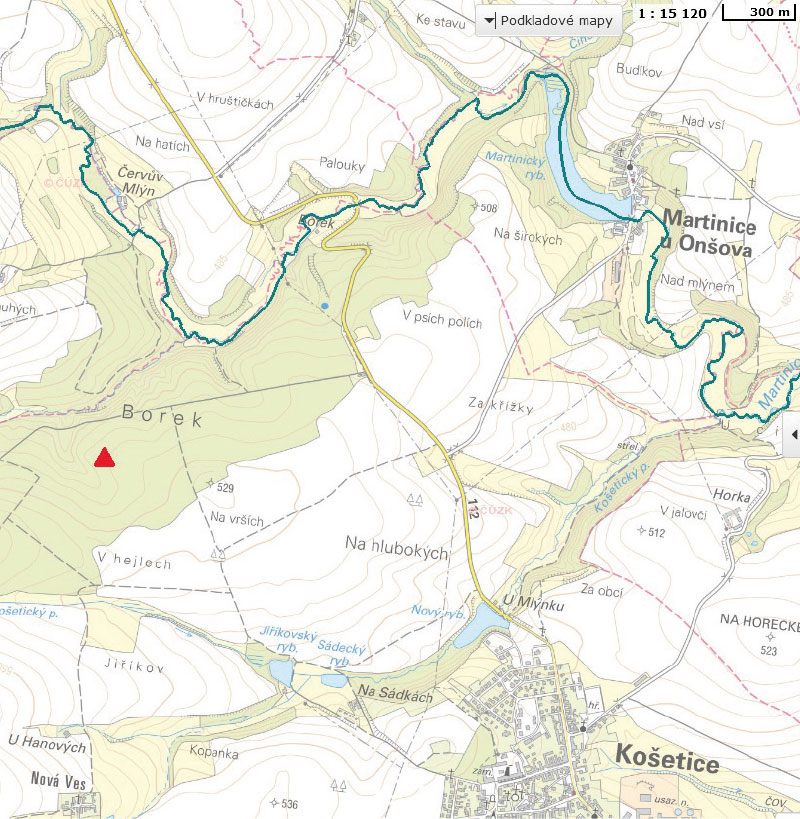
Fig. 3. Lesní stream site, Košetice (Source: HEIS TGM WRI)
In October 2020, monitoring of atmospheric precipitation in monthly campaigns was started at both sites (Tab. 1). In the case of insufficient rainfall, a two-month rainfall sample was used (sample volume required was 2,000 ml). Rain gauges were installed at the sites to capture bulk precipitation, and in the forest stand to capture throughfall precipitation. For the collection of precipitation for PAH determination, a rain gauge was made for a stainless steel container with a collection area of 52.4 cm2 (Fig. 4). The upper part of the rain gauges was equipped with a stainless steel bowl with holes so that the fall of coarse solid particles and insects did not get into the collected precipitation sample. A conifer (spruce in both cases) was chosen for the throughfall exposure, because precipitation was also collected in winter. The amount of precipitation recorded in individual campaigns was measured and compared with data on the total precipitation for the same period from the nearest climatological station of the Czech Hydrometeorological Institute (CHMI). At the same time, spot sampling of surface water from a nearby watercourse was carried out during the precipitation sampling. The average monthly flow for the Suchý stream was derived according to the flows at the nearest CHMI gauging station from the ratio of the areas of the given sub-basins. The average monthly flow of the Lesní stream was taken from regular measurements carried out by CHMI.
Tab. 1. Monthly precipitation amount and flows in sampling campaigns at the Bystřice and Košetice sites
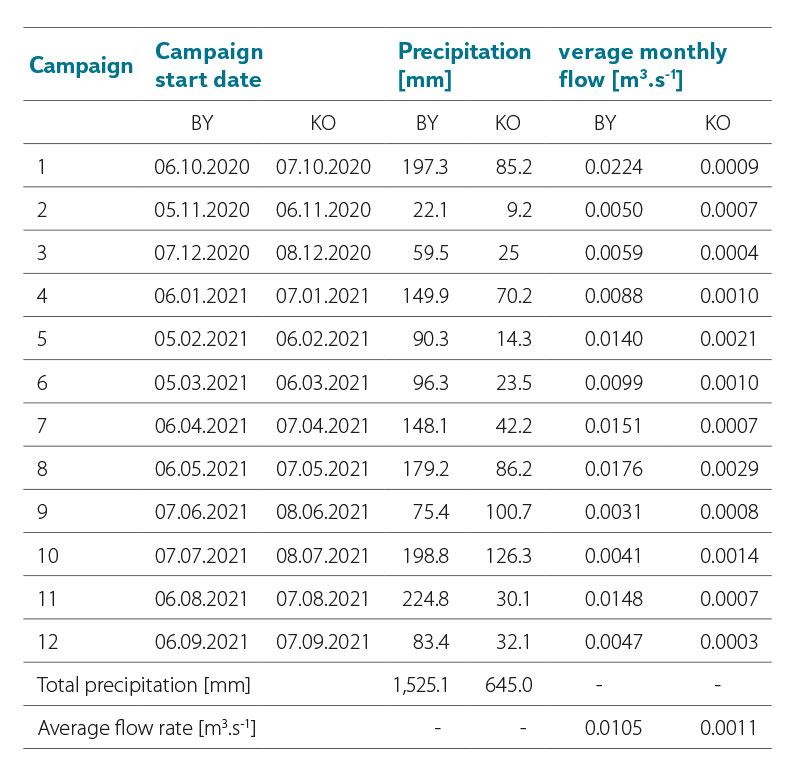
Fig. 4. Rain gauge for precipitation capture for PAH analysis
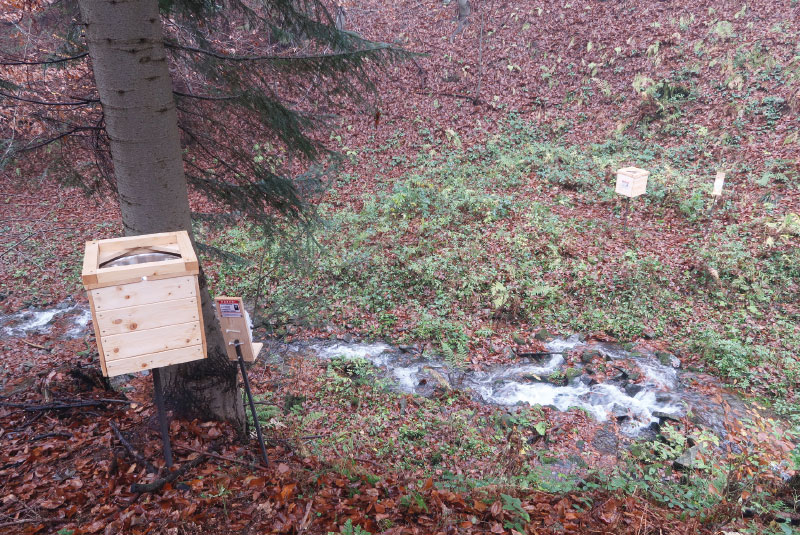
Fig. 5. Rain gauges for capturing bulk and throughfall precipitations at the Bystřice site (5th November 2020)
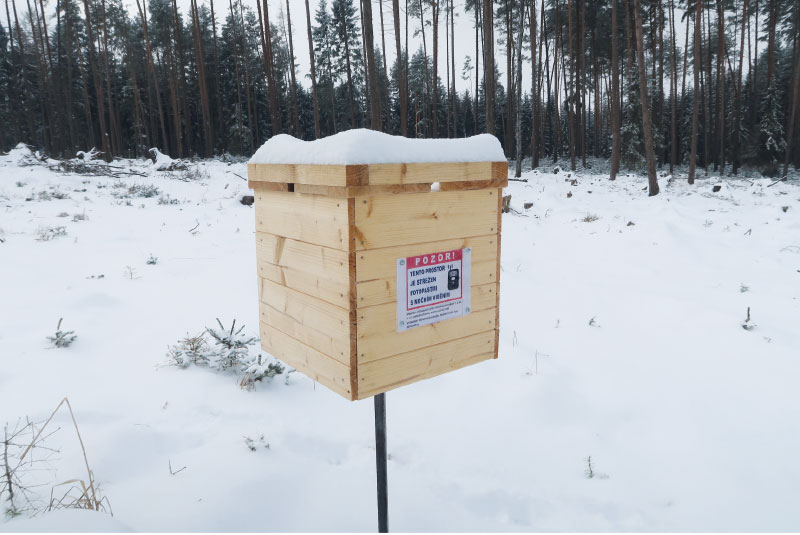
Fig. 6. Rain gauge for capturing bulk precipitation at the Košetice site (8th February 2021)
On the basis of field data, i.e., the amount of precipitation and the detected concentrations of monitored parameters of 15 PAHs in precipitation, an estimate of the total deposition for a given experimental basin was calculated according to the following formula:
Where: RS is the annual precipitation in a given basin
Sx amount of precipitation in a given month converted to the basin area
Cx concentration of the pollutant in the throughfall sample of a given month
The results of PAH concentrations in throughfall deposition were used in the calculation, which is considered the best possible estimate of total atmospheric deposition and is used in particular for determining the input of substances when balancing the circulation of substances in small basins [15].
The estimate of the annual substance ratio of watercourses for a given pollutant was calculated on the basis of the derived flow rate and the detected concentrations according to the following formula:
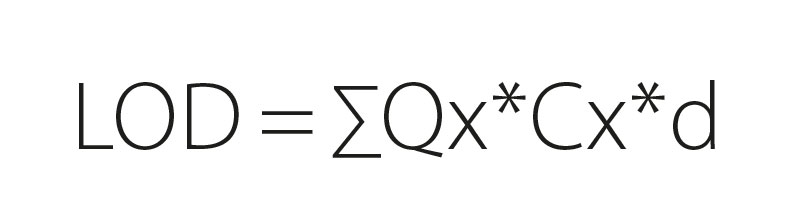
Where: LOD is riverine load
Qx average flow rate in the campaign
Cx concentration of the substance in the spot sample
D period
Values of concentration below the detection limit were not included.
Note: The usual procedure of using half the limit of determination was not chosen because the results of the two procedures show large differences.
RESULTS
In the following tables and graphs, the abbreviations listed in Tab. 2 are used for individual PAH compounds.
Tab. 2. Abbreviations used to designate individual PAH compounds
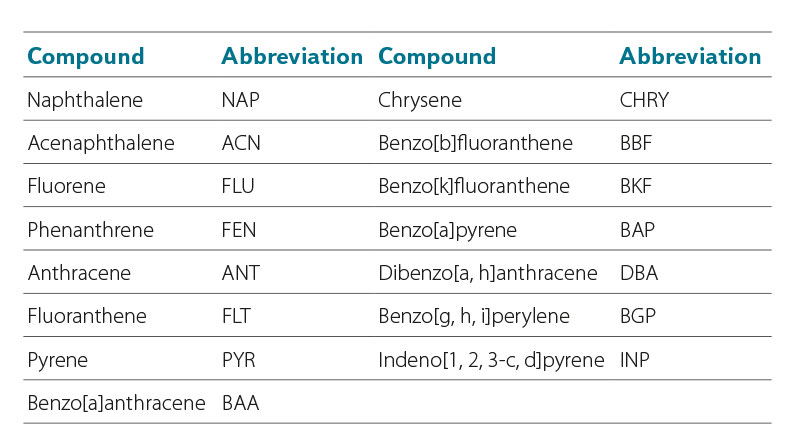
For information, the PAH results in precipitation and in surface water are compared with the limits of good surface water status according to Government Regulation No. 401/2015 Coll. [4]. These limits are environmental quality standards (EQS) expressed as an annual average value of AA-EQS and as the highest permissible concentration of MAC-EQS. Tab. 3 shows the result of the evaluation of surface water bodies where good chemical or ecological status was not achieved in terms of individual PAH indicators. The evaluation was carried out in 2016–2018 for the third river basin management plans. From the total of 1,118 surface water bodies, PAHs were measured in 53 to 65 % of them. The number of insufficient water bodies indicates the importance of these substances in terms of determining measures to achieve good surface water status.
Tab. 3. Assessment of surface water body status 2016–2018 in PAH parameters for the third planning cycle
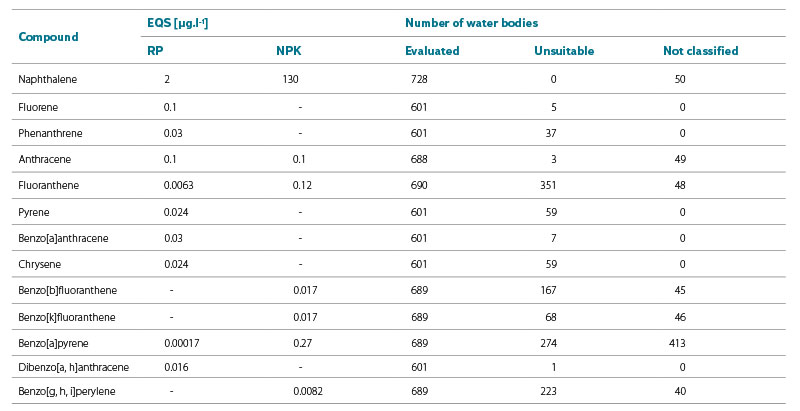
Tab. 4 and 5 show the results of PAH measurements in surface water and precipitation (bulk and throughfall) at Bystřice and Košetice. Values that are higher than the values of environmental quality standards for good surface water status are marked in red. The measurement results in individual sampling campaigns are compared with the MAC-EQS value, the calculated average annual value is compared with the AA-EQS value. The results are shown in Fig. 5–10. They show a high load of PAHs in precipitation at the Bystřice site.
Tab. 4. Results of measurements of individual PAHs in surface water and precipitation, Bystřice site
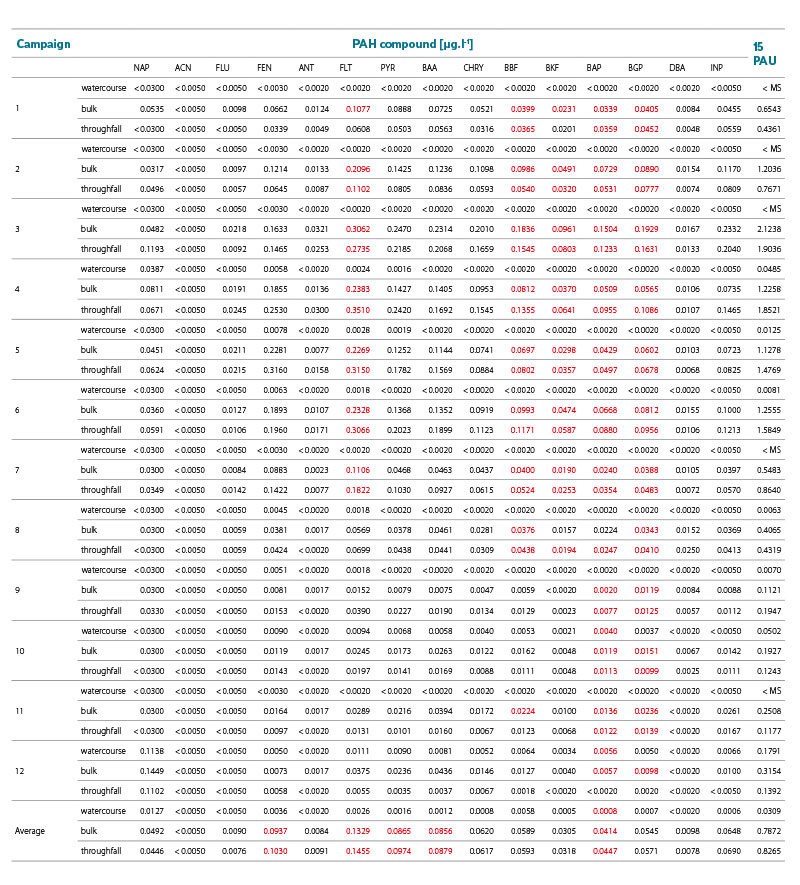
Tab. 5. Results of measurements of individual PAHs in surface water and precipitation, Košetice site
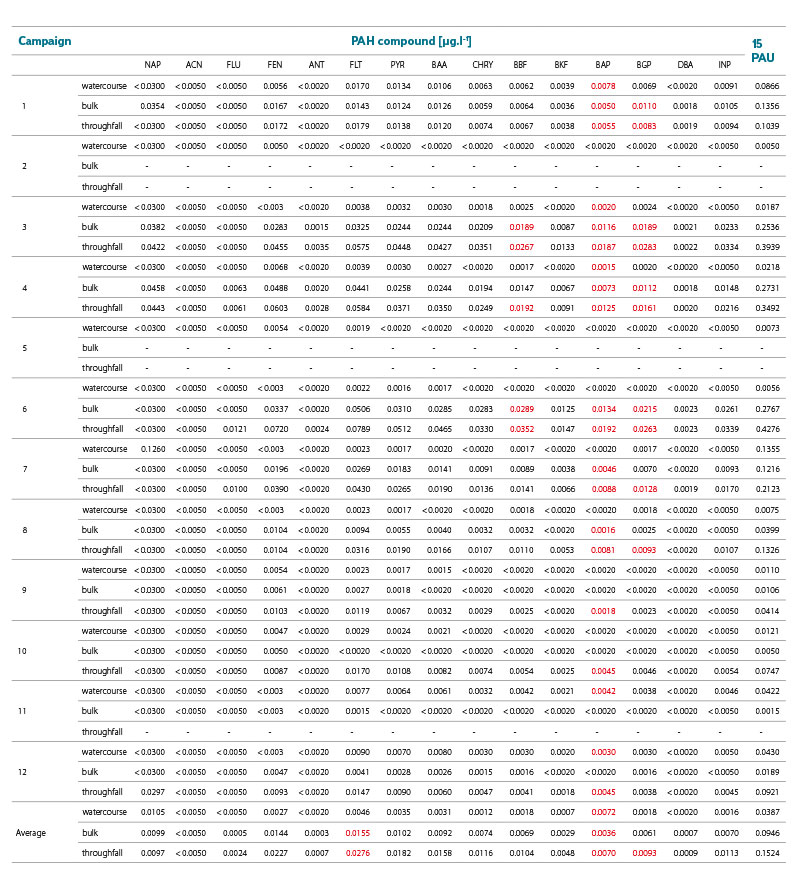
In Fig. 7, 8, 10, and 11, the trend in PAH pollution in winter and summer precipitation can be observed. The increase in concentrations in the winter period is most probably influenced by the local heating stations and meteorological conditions (temperature inversion?) during the colder part of the year. Of the individual PAH compounds, concentrations prevail in atmospheric precipitation in the Suchý stream – Bystřice in the following order: fluoranthene, pyrene, bezo[a]anthracene, phenanthrene, chrysene, and benzo[b]fluoranthene, and in the Lesní stream – Košetice in the following order: fluoranthene, phenanthrene, pyrene, benzo[a]anthracene, chrysene and benzo[b]fluoranthene.
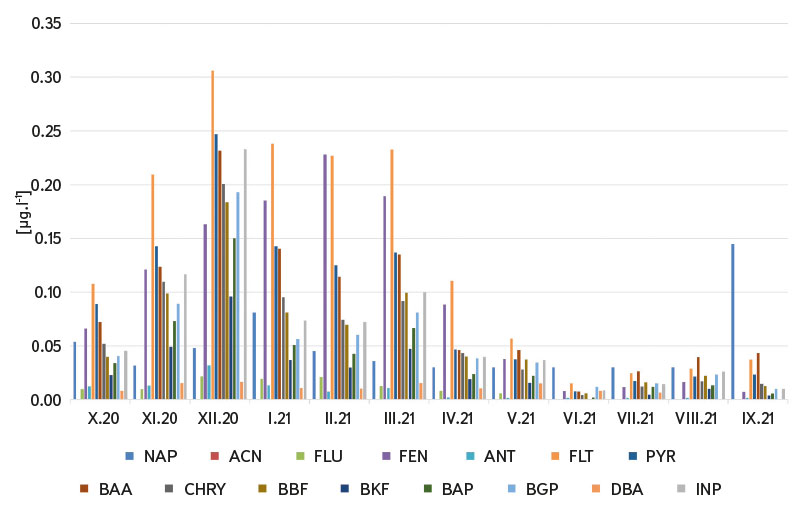
Fig. 7. Suchý stream, Bystřice – PAHs concentration in precipitation: BULK
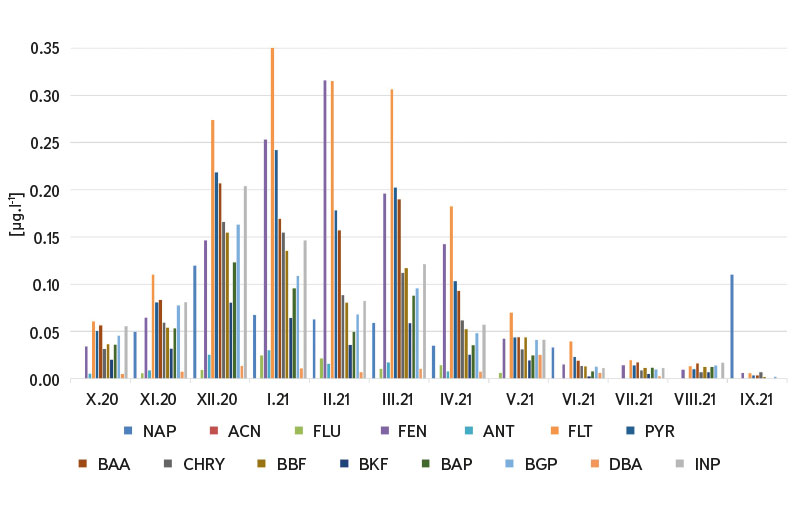
Fig. 8. Suchý stream, Bystřice – PAHs concentration in precipitation: THROUGHFALL
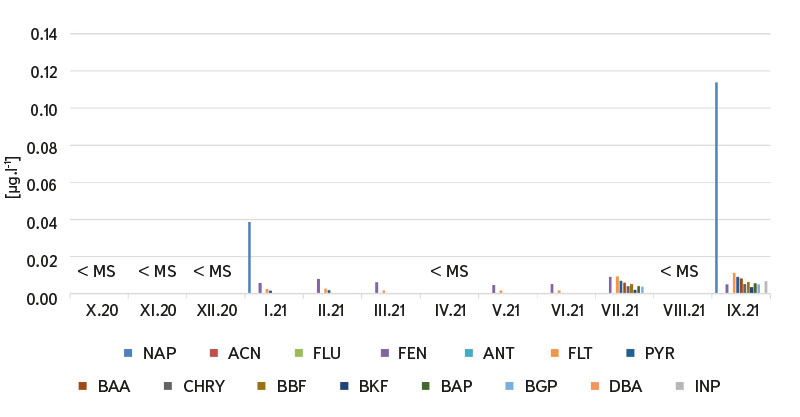
Fig. 9. Suchý stream, Bystřice – PAHs concentration in surface water
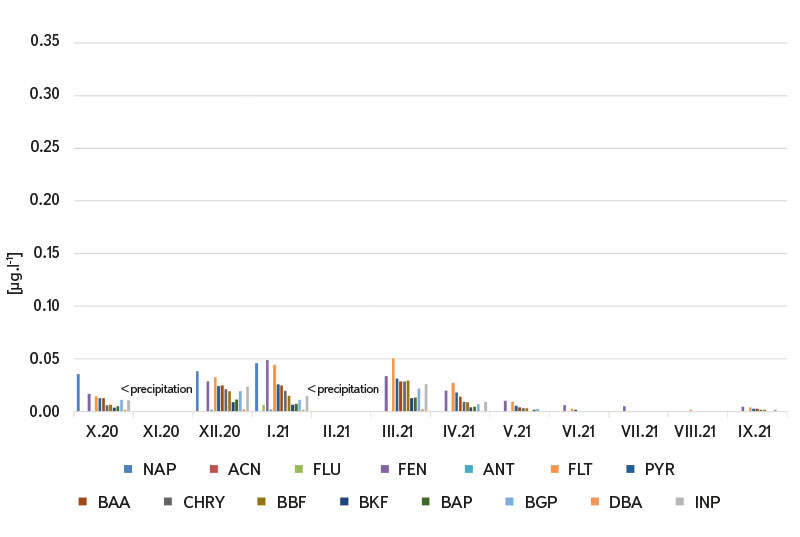
Fig. 10. Lesní stream, Košetice – PAHs concentration in precipitation: BULK
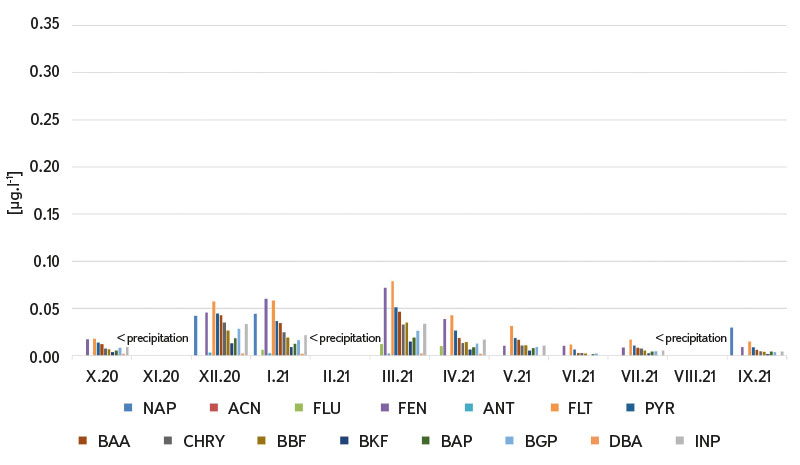
Fig. 11. Lesní stream, Košetice – PAHs concentration in precipitation: THROUGHFALL
Although high molecular weight PAHs are more easily sorbed onto fine dust particles in the air, it was not confirmed that the content of these PAHs was unequivocally predominant in the throughfall deposition in Bystřice; it was mainly observed in winter and spring. In contrast, at the minimally loaded site at Košetice, a higher PAH load in the throughfall deposition was the rule.
For comparison, the highest concentration of fluoranthene in throughfall precipitation in Bystřice was 0.306 µg.l-1 and in Košetice 0.076 µg.l-1.
The PAH representation in surface water is significantly lower compared to atmospheric deposition. The upper soil layers and the vegetation cover capture the majority of these non-polar organic substances, which are easily sorbed to fine dust and humus particles.
The highest concentration of monitored PAHs in the surface water of the Suchý stream in Bystřice (Fig. 9) was found for naphthalene (January and September). A larger spectrum of PAHs occurs as a result of large precipitation episodes. In winter, the concentrations of naphthalene, phenanthrene, fluoranthene, and pyrene dominate. In October, November, December, April, and August, PAH values were below the limit of determination. The highest concentration of naphthalene was found in the Lesní stream in Košetice (Fig. 12) in April. In winter, the concentrations of fluoranthene, phenanthrene, pyrene, and benzo[a]anthracene dominate. The composition of individual PAHs in surface water also correlates with higher precipitation episodes.
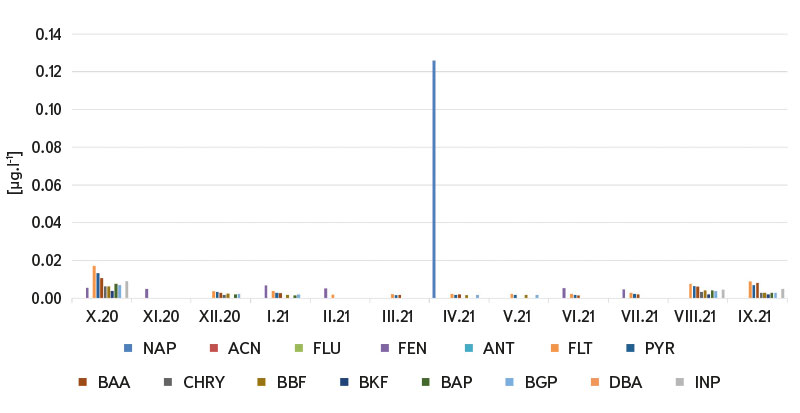
Fig. 12. Lesní stream, Košetice – PAHs concentration in surface water
Tab. 6 shows the results of the measured PAH values in the monitored solid matrices. These are average values from two to three measurements in the case of stream sediment and from three sites in each micro-catchment in the case of moss and humus. The detected PAH content is higher in sediment than in surface water. The content of the fine fraction of the Suchý stream sediment was very low because the morphology of the river bed, the slope of the mountain stream bed, and the dynamics of the flow do not allow the deposition of the fine fraction as in the case of the Lesní stream. Therefore, the content of PAHs in the river sediment of the Lesní stream is many times higher, despite the fact that it is a low-exposed reference area. At the same time, the PAH content in humus was more than three times higher at Bystřice than Košetice due to the high load from atmospheric deposition. The highly exposed PAH load of the Bystřice site was also manifested in red-stemmed feathermoss, which receives nutrients for its growth exclusively from the atmosphere (which is why it is used as a suitable marker of atmospheric load). The analysed parts of the moss represent an approximately three-year period of PAH exposure. The concentration ratio of the amount of PAH between the two monitored sites in moss and humus is approximately the same (3.0 and 3.5, respectively).
Tab. 6. Indicative comparison of PAH concentrations in other monitored matrices in 2020 and 2021
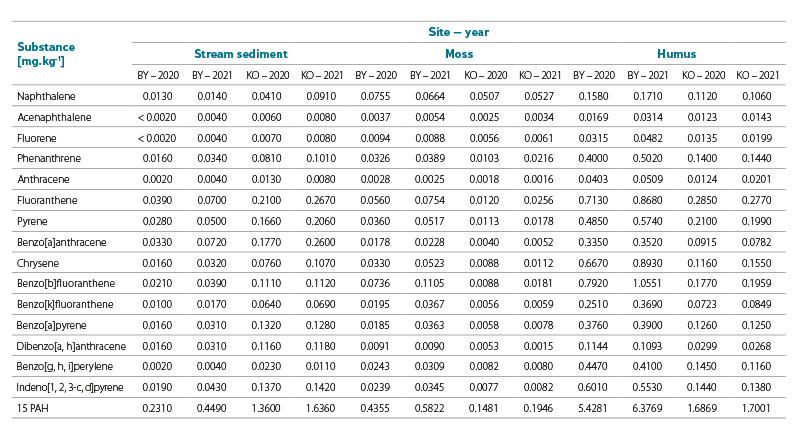
Tab. 7 and Fig. 13 provide a summary of the calculated atmospheric deposition and substance ratio both absolutely and relatively per unit area at both experiment sites.
The calculated results confirm (Tab. 7) that in the case of PAH, rainfall pollution is many times higher than surface water pollution (Fig. 13). The Ostrava-Třinec industrial agglomeration is one of the areas with the highest concentration of PAH in the Czech Republic. This is also confirmed by the results from Bystřice.
Tab. 7. Calculation of atmospheric deposition and riverine load at pilot sites
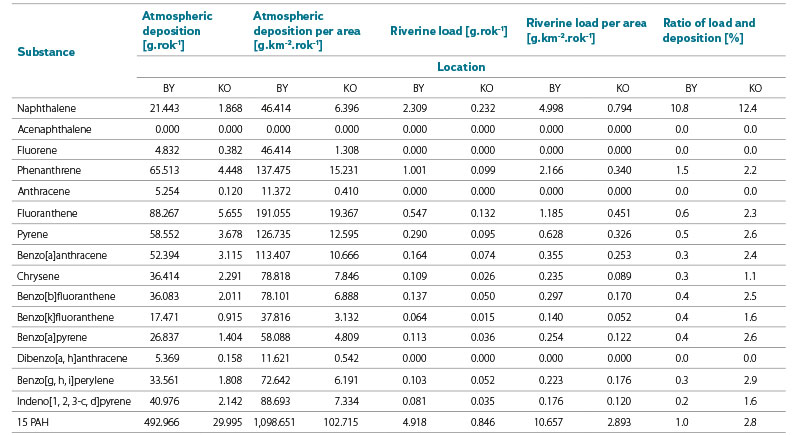
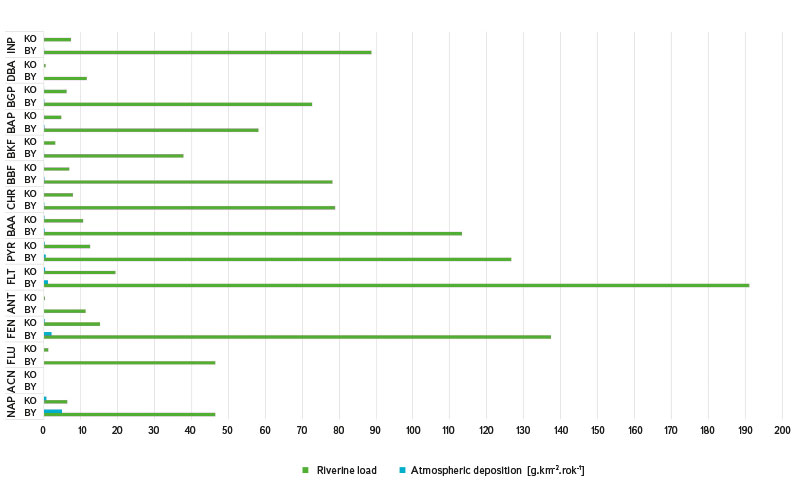
Fig. 13. Calculation of atmospheric deposition and riverine load per area
For the concentrations of individual PAH compounds in the atmospheric deposition per area (g.km-2.year-1), the following order applies:
Bystřice:
FLT > FEN > PYR > BAA > INP > CHR > BBF > BGP > BAP > FLU > NAP > BKF > ANT > DBA > ACN
Košetice:
FLT > FEN > PYR > BAA > CHR > INP > BBF > NAP > BGP > BAP > BKF > FLU > DBA > ANT > ACN
For the concentrations of individual PAH compounds in the ratio of substance to area (g.km-2.year-1), the following order applies:
Bystřice:
NAP > FEN > FLT > PYR > BAA > BBF > BAP > CHR > BGP > INP > BKF > ACN, FLU, ANT, DBA
Košetice:
NAP > FLT > PYR > BAA > FEN > BGP > BBF > BAP > INP > CHR > BKF > ACN, FLU, ANT, DBA
DISCUSSION AND CONCLUSION
Field measurements carried out in selected forest micro-catchments of Bystřice and Košetice confirm that pollution of precipitation by polycyclic aromatic hydrocarbons is many times higher than surface water pollution. The contribution to PAH by atmospheric precipitation is more significant than by surface water, which is confirmed by, for example, Lipiatou [16]. Significant seasonal variation in PAH concentrations in precipitation with maxima in winter was recorded. PAHs are removed from the atmosphere by dry and wet deposition. The range of concentrations is directly dependent on meteorological conditions. Seasonal changes in PAH concentrations show a maximum in winter and a minimum in summer. Maxima in the cold season of the year in connection with the frequent burning of fossil fuels and atmospheric conditions may not always lead to an increased PAH content in the rivers. In summer, forest fires are also a significant source of PAHs in connection with increasing climate change [17]. Higher temperatures contribute to more effective oxidation by atmospheric trace gases (NOX, SO2, O3), so that their degradation takes place faster in summer than in winter. Their regional distribution is dependent on local sources, with the main sources being fossil fuel combustion processes, domestic heating, and vehicle transport.
The PAH content in precipitation depends on their solubility in water. PAHs with a low molecular weight are soluble within mg.l-1, while higher PAHs are soluble within ng.l-1. PAHs with a lower molecular weight are found in the atmosphere on solid particles as well as in the gas phase; with increasing molecular weight, PAHs are more sorbed on solid particles and only a small part is in the soluble fraction.
Gas-phase PAHs become part of wet atmospheric deposition through interfacial gas-liquid exchange in the below-cloud washout process, while PAHs associated with solid particles are more effectively washed out by intra-cloud washout processes as a consequence of diffusion, impaction, and entrapment [18].
Particles with bound PAH compounds from combustion processes can be transported long distances in the atmosphere and thus reach areas without obvious sources. This long-range transport mechanism depends on the particle size of the atmospheric aerosol. Aerosol particles of smaller dimensions (< 1 mm), which are not effectively removed from the atmosphere by dry and wet deposition processes, remain in the atmosphere for a longer time and may therefore be the reason for their presence in remote areas. Larger atmospheric aerosols (> 5 µm) are more effectively removed by precipitation and are deposited closer to their sources, which is the case of the Bystřice site in the Suchý stream basin.
The amount of Σ15 PAH atmospheric deposition at the Bystřice site was calculated at 1,098.7 g.km-2.year-1; at the Košetice site it is 10 times lower at 102.7 g.km-2.year-1. Fluoranthene (18 %), phenanthrene (13 %), and pyrene (12 %) contribute the most to this deposition. For comparison with already published data, the deposition was converted to ng.m2.d-1: 3,010 ng.m2.d-1 (BY) and 102 ng.m2.d-1 (KO). In rural areas, atmospheric deposition (bulk) is reported to be 38–2,000 ng.m2.d-1, in urban areas 36–20,000 ng.m2.d-1 [17]. When normalizing the amount of Σ15 PAH atmospheric deposition at the Bystřice site to the amount of precipitation at the Košetice site, the result is that the load of polycyclic aromatic hydrocarbons in Bystřice is 4.5 times higher than at the reference site of Košetice.
The top soil layer and vegetation cover sorb PAHs. PAHs enter surface waters through erosion. The Σ15 PAH concentration in the Suchý stream in Bystřice was 0.026 ± 0.049 mg.l-1, in the Lesní stream in Košetice it was 0.033 ± 0.038 mg.l-1. Riverine load from the Suchý stream micro-catchment thus accounted for only 1 % of the atmospheric deposition by wet deposition in Bystřice and 2.8 % from the Lesní stream micro-catchment in Košetice.
Due to their extremely low volatility and low solubility in surface water, high molecular weight PAHs occur in very low concentrations. However, their contribution to surface waters is significant during higher rainfall episodes, when erosion and runoff from paved areas are applied. The accuracy of the balance of PAH riverine load through surface waters is affected by:
- the time of surface water sampling in relation to precipitation in the previous period,
- proportion of fine particles in river sediment,
- flow rates at the time of sampling, when the fine fraction of sediment in the water column rises at higher flow rates.
Given that only some point samplings of surface water in the experimental micro-catchments of the Suchý stream and Lesní stream were carried out immediately after a rainfall-runoff event with possible erosional wash, the actual proportion of PAH riverine load through surface water to the balance of atmospheric deposition through wet deposition will probably be higher than the above values 1 % and 2.8 %. Further clarification of the effect of PAH atmospheric deposition on surface water quality will require more research, also due to the significant proportion of surface water bodies not achieving good chemical status in most PAH indicators.
The origin of PAHs can be inferred from the ratio of fluoranthene to pyrene [e.g., 19]. If this ratio is greater than 1, the origin are combustion processes; if it is lower than 1, the origin are petrochemical products. Both at the Bystřice and Košetice sites, this ratio was greater than 1. Specifically, in the total wet deposition (bulk) it was 1.6 in Bystřice and 1.5 in Košetice. In the case of throughfall deposition, it was almost the same: 1.5 and 1.55 in Košetice. In the colder part of the year, this ratio in Bystřice was slightly higher (bulk 1.7) than in summer. In the Suchý stream, the ratio of fluoranthene to pyrene was 1.4, in the Lesní stream it was 1.3. Similar ratios were confirmed in solid matrices except for humus: in the Suchý stream basin, the ratio was 1.5, but in the Lesní stream basin it was 2.4. In red-stemmed feathermoss, the ratio of both polyaromatic hydrocarbons was 1.5 in Bystřice while in Košetice it was a slightly lower at 1.25.
A more detailed description of the representation of PAHs in the monitored matrices and the links between the pollution of individual components of the environment is available on the project’s website [20].
In autumn 2022, the European Commission published a draft amendment to Directive 2008/105/EC, in which the AA-EQS environmental quality standard for fluoranthene is significantly tightened. The issue of environmental and water pollution by polycyclic aromatic hydrocarbons is thus gaining importance.
Acknowledgements
This article was supported by the Technological Agency of the Czech Republic grant SS01010231 “Impacts of atmospheric deposition on the aquatic environment with consideration of climatic conditions”.
This article was translated on basis of Czech peer-reviewed original by Environmental Translation Ltd.