ABSTRACT
The aim of the study, the results of which are presented in this article, was to assess the possibility of simplifying treatment and stabilisation procedures of sewage sludge from small municipal sources of pollution (domestic and small WWTPs up to about 1,000 EP) at the place of their origin and their subsequent use through extensive composting. The results demonstrated the benefit of the application of composts from a material base containing sludge from small WWTPs in increasing the production of the monitored types of vegetables. However, especially with lettuce, there was a higher transmission of selected risk elements. We therefore do not recommend the use of composts with sludge for growing leafy green vegetables. In contrast, this risk did not arise with fruit and vegetables. For practical use, it is still necessary to assess the rate of transfer of other pollutants, such as drug residues and microplastics.
INTRODUCTION
Sludge from wastewater treatment represents a valuable source of nutrients, but at the same time contains a number of hazardous elements, organic pollutants, and other substances. In its raw state, it is loaded with relatively significant microbial contamination. As part of the principles of the circular economy, the possibilities of limiting its contamination, as well as its stabilization and processing into substrates that can be used in agriculture, or in the care of green areas and greenery in general, have been studied for a long time. Restrictions on the use of sewage sludge in European countries are presented in the paper [1]. A summary overview of the restrictions on the application of sludge in agriculture, which is based on the valid European directive from the 1980s, and an overview of the management of sludge in EU member countries as of 2019 is given in Hudcová et al. [2]. The direct use of sludge and indirect use after processing by composting is very different in the EU member states and corresponds also to local conditions and how individual countries approach the risks of using sludge on land. The main danger associated with the application of sludge on agricultural land is the potential long-term accumulation of toxic substances [3], which can then be taken up by crops. Composting is one of the options for pre-treatment of sludge and other waste from water treatment processes, which should bring about modification of their properties [4, 5].
Composted sludge is a source of a whole range of nutrients for plant growth (e.g. phosphorus, nitrogen), organic matter, and microorganisms useful for the soil. Its use reduces the consumption of fertilizers and pesticides and improves the physical and biological properties of the soil; however, at the same time, excessive application can lead to the accumulation of heavy metals in the surface layers of the soil [6]. During composting, which is the aerobic biological decomposition and stabilization of organic substrates, microbial populations develop which cause numerous physico-chemical changes in the mixture. Composting can reduce the volume of the mixture by 40–50 %, effectively destroy pathogens through the metabolic heat generated by the thermophilic phase, degrade large amounts of hazardous organic pollutants, and provide a final product that can be used as a source of organic matter, slow-release nutrients, and trace elements for the soil [7–12]. Sewage sludge is often composted before application to the soil, also with the aim of reducing the availability of heavy metals, as this process results in the mineralization of organic compounds that control the availability of cations to plants [13].
There is a general consensus in the scientific literature that aerobic composting processes increase the complexation of heavy metals in organic waste residues and that metals are strongly bound to the compost matrix and organic matter, limiting their solubility and potential bioavailability in soil. The most strongly bound is Pb, the weakest are Ni and Zn, Cu and Cd, which show moderate sorption characteristics. Metal availability decreases with composting time and maturation [14].
The aim of the study, the results of which are presented in this article, was to assess the possibility of simplifying treatment and stabilization procedures of sewage sludge from small municipal sources of pollution (domestic and small WWTPs up to about 1,000 p.e.) at the place of their origin and their subsequent use through extensive composting. The result should also be to assess the benefits and risks when applying the resulting composts for growing selected types of crops (vegetables) on a community scale. The study was thus intended to supplement information for decision-making; namely, whether it is possible to consider a different method of local processing and utilization of sludge from the mentioned types and sizes of WWTPs than the standard procedure consisting of regular transfer to a larger WWTP with sludge management.
METHODOLOGY
For the presented study of the effect of composting sludge and waste from reed bed plants on the transfer of nutrients and pollutants to selected types of vegetables, sludge and waste from domestic and small WWTPs of two basic technologies were used: activation WWTPs and reed bed plants. A more detailed description of treatment plants that are the subject of long-term research, as well as an overview of conclusions from detailed analyses of their sludge, are given by the authors in other publications [15, 16].
Material and composition of experimental composts
With regard to the simulation of the possible actual process of treating sludge from domestic and small WWTPs as part of composting with other organic materials from smaller sources (domestic biowaste, community biowaste), we chose to carry out composting in plastic composters with a volume of several hundred litres (Fig. 1) and in small trapezoidal piles of material of a similar volume, covered with a foil.
Fig. 1. Composting containers used for community composting (left); one of the final compost mixtures for the pot experiments (right)
In the first year, two composts were created in plastic composters with a volume of 500 litres: one with sludge from the domestic activated WWTP (marked K-A) and the other with sludge from the reed bed plant (marked 1 K-K). In the case of sludge from the reed bed plant, one more experimental pile of material was prepared in the form of a trapezoidal pile under foil, with a volume of 4,000 litres. The compost was marked as 2 K-K. Layers of sludge in a total volume that corresponded to the principles established by the SN regarding composting (i.e. a maximum sludge content of up to 40 % of the pile) were interspersed with layers of grass from mowing, layers of chips from processed wood matter and, in the case of a reed bed plant, also with additional layers of macrophyte vegetation from reed bed filters of the plant (reed with an admixture of iris and great manna grass). The ratio of input materials corresponded to the requirement for the recommended C/N ratio, which is reported in the range of 20 to 30/1 [17, 18], while the addition of green and wood matter during sewage sludge composting aimed to increase the C/N ratio [19].
In the second year, composts were established in trapezoidal piles covered with PE black impermeable foil with a volume of about 300 litres using sludge from two sizes of reed bed plants (domestic – compost K-3 and municipal – compost K-4). The layers were placed as follows: bottom layer 10 cm – wilted grass, above it a 5 cm layer of sludge from the reed bed plant (dry sludge about 14 %), above that a 15 cm layer – wilted grass, then a 5 cm layer of sludge from the reed bed plant (dry sludge about 14 %) and the upper layer consisted of a 10 cm layer of wilted macrophytes. The description of the municipal reed bed plant is given in Rozkon et al. [20].
During the composting process, the ambient air temperature and the temperature and humidity of the environment in the compost were monitored.
During composting, mixed samples of the resulting compost were taken to analyse the current level of microbiological contamination (enterococci, faecal coliform bacteria) and the content of nutrients and macroelements (N, P, K, Ca, Mg, Na) and heavy metals (Al, As, Cd, Cr, Cu, Fe, Hg, Mn, Ni, Pb, Zn). Dry matter, loss by annealing, and the content of culturable microorganisms at 22 °C were also monitored. Sampling took place for composts established in the first year after four and twelve months from establishment; for composts established in the second year after four, seven and twelve months from establishment. The length of the composting process corresponded to experience with the course of extensive small-volume (domestic, community) composts, which are characterized by a longer period of maturation and stabilization.
Assessment of the effect of composts on the production of selected crops
Assessment of the effect of composts on the production of selected crops was carried out using pot experiments. Among the studied crops (also on the basis of research), crops representing different types of vegetables (leaf, fruit) were selected: lettuce (Lactuca sativa L.) – Maralus variety (Fig. 2) and tomato (Lycopersicon esculentum Mill.) – Tornado F1 variety (Fig. 3). Lettuce is one of the most commonly consumed raw leafy vegetables [24] and is classified as a plant sensitive to heavy metals [21–23]. Tomatoes are the second most important vegetable in the world after potatoes; in 2016, annual global production was 177 million tons grown on almost 4.8 million ha of land [24].
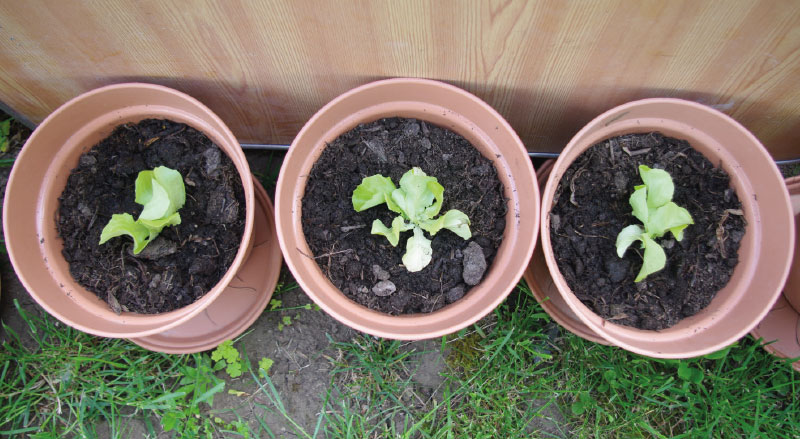
Fig. 2. View of part of the containers from the lettuce planting pot experiments; mixing of compost with soil in the upper layer of about 5 cm is evident
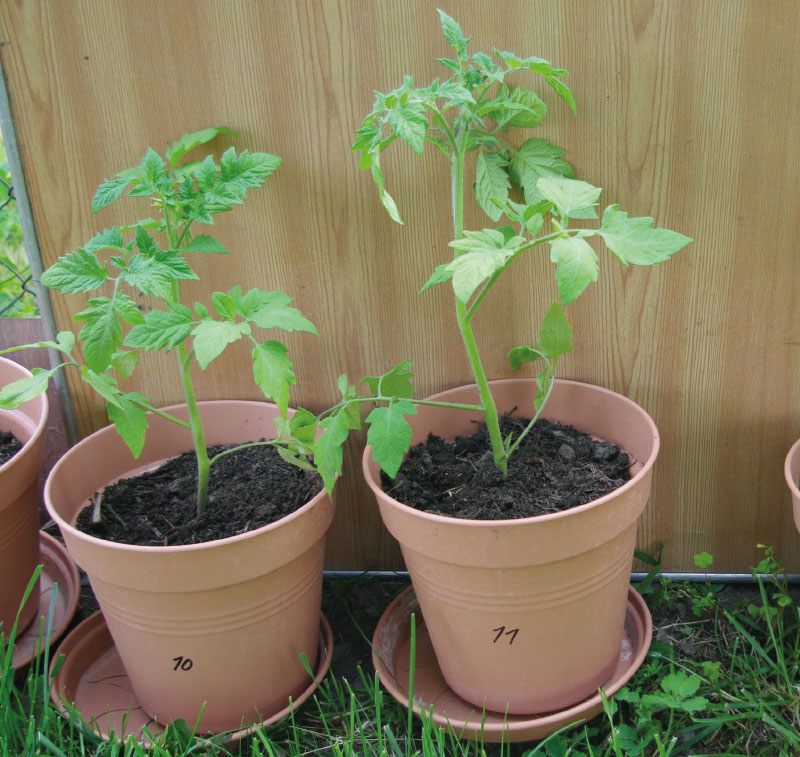
Fig. 3. View of part of the containers from the tomato planting pot experiments
Pot experiments for growing selected types of vegetables
Pot experiments were designed using the same five-litre plastic pots with a surface area of 0.031 m2. All sets were placed in the same location and under the same conditions. Two (experiment 1 year) or three repetitions (experiment 2 year) were prepared for each variant of the substrate (soils, composts, mixtures of soils and composts). Substrates were chosen to include comparative soils – fertile garden soil (chernozem – Hustopesko region), designated as ZZ in the experiments, and degraded eroded field soil (chernozem – Hustopesko region), designated as EZ in the experiments. Then there were mixtures of these soils with composts, and only composts. Soils and composts were homogenized by mixing before being filled into containers, and portions were subsequently placed into individual containers. The materials were prepared in a rainless period with the following humidity levels: first year experiment – soils about 94 % dry matter, composts about 73 %; second year experiment – soil about 94 % dry matter, composts about 74 %. Mixtures of field soil with composts were prepared in such a way that the proportion of compost corresponded to the theoretical field dose of 80 tons of compost per hectare. After conversion, it was 260 g in one five-litre container. The amount of soil in the mixture weighed about 3 kg. The proportion of compost in the mixtures was about 8 %. The compost was always mixed with the soil individually when filling each container, incorporating it into the upper layer of the soil to a depth of about 5 cm (Fig. 2).
Processing and analysis of vegetable, soil, and compost samples
Lettuce was harvested about one month after planting the seedlings (May), at the time of full maturity of the lettuce heads before their transition to the phase of flower formation (Fig. 4). Tomato plants were planted on the same dates as the lettuce. Harvesting of tomato fruits took place from the first appearance of ripening fruits (July) until the end of production of ripening fruits (September, October). Lettuce samples were dried at room temperature, finely crushed, and homogenized. Harvested tomato fruits were weighed fresh and stored in a freezer. At the end of the harvest, all fruits from a given plant were mixed, processed in the laboratory into a homogeneous mixture, and freeze-dried to take sample portions for analysis. To determine Al, As, Cd, Cr, Cu, Ni, Pb, Na, K, Ca, Mg, Fe, Mn and Zn, each sample (about 1g) was mineralized in a Teflon container by MLS-1200 MEGA device using 3 ml of concentrated HNO3 and 1 ml of 30% H2O2. The containers were sealed for the mineralization cycle to take place. After cooling, the contents of the container were transferred into a 100 ml volumetric flask. The determination of Al, As, Cd, Cr, Cu, Ni, and Pb was carried out by the method of atomic absorption spectrometry – electrothermal atomization (AAS-ETA) on a PERKIN ELMER AANALYST 600. The determination of Na, K, Ca, Mg, Fe, Mn and Zn was carried out by the method of flame atomic absorption spectrometry (AAS-flame) on a PERKIN ELMER AANALYST 400. The calibration curve method was used to determine the content of individual metals. The correctness of the determined concentrations was verified using the simultaneous analysis of internal and reference material. Determination of Hg was carried out on an AMA-254 mercury analyser, calibrated according to the manufacturer’s manual. Approximately 0.1 g was weighed from the pre-treated sample. The Hg content determined always corresponded to the average of two to three simultaneous determinations. The correctness of the determined concentrations was verified using the simultaneous analysis of internal and reference material. Homogenized samples of composts and soils were freeze-dried and then processed in a manner identical to the processing of biomass samples. Total phosphorus was determined using the cuvette test LCK 348 (HACH-LANGE) on a DR 3900 spectrophotometer with a tungsten lamp (Vis). Total nitrogen was determined by the modified Kjeldahl method according to SN ISO 11261.
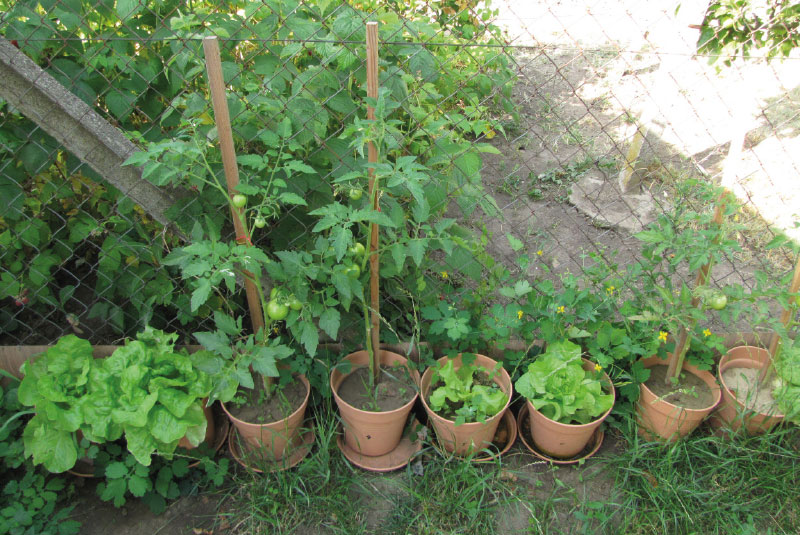
Fig. 4. View of part of the planting pot experiment containers; in the left part, lettuce heads and tomato plants with fruits in a substrate with compost, in the right part in a substrate without compost
Assessment of phytotoxicity of composts using the seed germination test
In the case of using sludge from small sources (domestic and small municipal WWTPs) for horticultural and agricultural purposes, the main interest of the user is also to reduce the contamination of the resulting sludge and to ensure that the substrates used that contain sludge or compost do not pose a health risk and a danger to the environment in terms of toxicity. This fact can be verified, for example, by phytotoxicity tests or earthworm escape tests [25]. Phytotoxicity tests exist in the form of directives issued by major environmental agencies, such as the US EPA (United States Environmental Protection Agency), OECD (Organization for Economic Co-operation and Development), ISO (International Standards Organization), ASTM (American Society for Testing and Materials), and others. Papers from 2011 and 2019 give an overview of phytotoxicity tests [26, 2].
The seed germination test, which was chosen for our study, is a method of evaluating the intensity of decomposition of organic materials and the maturity of the resulting compost, which was developed at the Crop Research Institute Prague for use in composting practice. It is a biological method of evaluating the phytotoxicity of a sample leachate using the germination index of a sensitive plant – garden cress (Lepidium sativum) [27].
The resulting germination index can be obtained from the following equation:
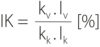
where:
kv is the germination rate of the sample [%]
kk control germination [%]
lv average root length of the sample [mm]
lk average root length of control [mm]
At values up to 50 %, the index states that the compost is unusable for direct application, from 60 to 80 % it gives the possibility of application with a certain risk of damage to sensitive plants, and at values of 80 % and higher it declares mature compost. If the germination index is between 60 and 80 %, it can be said that the compost is in the conversion phase and has the best fertilizing effect. Above 80 %, this effect decreases, and the influence of humus is stronger, which means that nutrients are more bound. The release of N and P is slower and there is no leaching of nutrients into groundwater [28].
Statistical analyses were performed using available tools in MS Office Excel 2016 and SW R-4. 3. 2. for Windows using ANOVA analysis of variance after pre-screening the data sets for standard distribution. The choice of procedure and statistical methods corresponded to the procedures that were used in a similar experiment focused on the effect of addition of sewage sludge composts to substrates for horticultural purposes [29]. In the case of pot experiments, the evaluation was carried out by calculating statistical characteristics for individual variants of substrates and crops from two (first year) and three repetitions (second year).
RESULTS AND DISCUSSION
Composition and contamination of used soils and composts
The contents of heavy metals and arsenic in the soils used in the experiments in both years did not exceed the preventive and indicative values according to National Decree No. 153/2016 Coll. Of the composts used in the first year, all composts exceeded both the proposed limit value for Cu in the framework of the EU technical report [30] and the given national standard. The composts also exceeded both limit values for Zn, and in the case of K-A compost, the limit value for this element was also exceeded according to SN 465735 [31]. K-3 and K-4 composts used in the second year did not exceed any of the limit values proposed within the EU and given by the SN 465735 standard. Lower concentrations of Cu and Zn in these composts (on average 218 mg/kg Zn and 65.9 mg/ kg Cu compared to the values of 1,016 mg/kg Zn and 386 mg/kg Cu in the composts in the first year) were probably caused by the lower proportion of used sludge in the input mixture for composting. Sludge load can be affected by the connection to the sewage system, which also brings rain wash rich in these metals due to the corrosion of roofing materials.
Regarding the assessment of microbial contamination, it was carried out using standard analytical methods for the determination of indicator organisms (Salmonella sp., enterococci, thermotolerant coliform bacteria) in the input sludge and in the resulting substrates from composting. Microbial contamination of sewage sludge from domestic WWTPs ranged from 2 103 to 4.2 103 KTJ/g of dry matter of samples in the case of enterococci and 1.6 104 to 6 104 KTJ/g of dry matter of samples in the case of thermotolerant coliform bacteria. The amount of thermotolerant coliform bacteria in the sludge from the municipal reed bed plant was in the range of 1 105 to 2 106 KTJ/g of dry matter and the number of enterococci in the range of 1 104 to 6 106 KTJ/g of dry matter. Microbial contamination in fresh substrates from composts before their use in pot experiments was zero for composts K-3, K-4 and 1 K-K (zero detection of KTJ per gram of dry matter) for both indicators. For composts 1 K-A and 2 K-K in tens of KTJ per gram of dry matter for enterococci and in lower hundreds of KTJ per gram of dry matter for FC. For all composts, it would be acceptable when assessed with the limits listed in the SN as “Composting”. The presence of Salmonella sp. was not detected even in the input sludge.
Phytotoxicity test results
Mixed samples were taken from the set of composts established in the second year (K-3 and K-4) and supplemented with a control sample (seeds germinated only on distilled water) for phytotoxicity seed germination test. Compost samples were already stabilized, they did not show changes in microbial contamination and content of heavy metals and macroelements. The garden cress test was performed in two dilutions, namely 5 and 10 dry weight (%). For each sample, 10 Petri dishes with 8 seeds were used, for a total of 80 seeds. After 24 hours, the number of germinated seeds in each Petri dish was determined and the lengths of all roots were measured.
Some vegetative responses, such as the seed germination test or the elongation of root and seedling growth, are commonly used to assess the excess toxicity of organic and inorganic compounds in various substrates [32]. The average germination rate in our experiment was found to be 7.5, 7.5, 7.7, and 7.8 seeds out of 10 for individual prepared mixtures of composts and soils, and 7.8 for the control set. ANOVA analysis showed that the null hypothesis of equality of mean values of the mixtures and the control set could not be rejected at the significance level of = 0.05 (p < 0.05). The spread of root lengths between the minimum and maximum values for the prepared mixtures was generally in the same interval of 4.0 to 9.0 cm with average values of 6.6, 6.8, 6.2, and 5.8 cm. The smallest average length (5.4 cm) was achieved by sprouts from the control set. The results of the phytotoxicity test show that the mixtures used were stabilized, without a negative impact on the germination of garden cress seeds. The IK index values ranged from 107 to 118 for all four mixtures.
Effect of compost application on change in yield of useful parts of crops
In the case of containers with lettuce seedlings, the difference in the weight of the above-ground part (leaves) of the grown head of lettuce without damaged and dry leaves on the edge was monitored. In both years, a statistically significant difference in fresh biomass weight was demonstrated (ANOVA, alpha level 0.05). In the first year, the average weight of the fresh head of lettuce was 15.7 g when using the EZ soil and 61.2 g when using the ZZ soil. In containers with 100% compost substrates, the average weight of fresh heads of lettuce was 77.5 g (1 K-K), 82.8 g (1 K-A), and 99.1 g (2 K-K). An 8% admixture of compost to poor-quality soil contributed to a substantial increase in yield. The average weights of fresh heads were 104 g (mixture with 1 K-A), 105 g (mixture with 1 K-K), and 95.2 g (2 K-K). This is an increase of up to 85 % compared to EZ soil, and 36 to 41 % compared to high-quality ZZ soil. An experiment in the second year confirmed these results. The average weight of a fresh head of lettuce when using EZ was 81.4 g, i.e., much higher than in the first year. However, the EZ used in this year contained 38 % more organic matter compared to the EZ used in the first year. In containers with 100% compost substrates, the average fresh weights of lettuce heads were 154 g (K-3) and 108 g (K-4). When using compost K-3, the average yield increased by 47 % when using compost K-4 by 25 %. An 8% admixture of composts to the soil meant an increase in average yields by 27 % (compost K-3) and by 14 % (compost K-4) to values of 111 g (K-3) and 95.3 g (K-4). Fig. 4 shows the difference in the size of the lettuce heads as a result of the use of compost in growing substrates.
In the case of tomato plants, the influence of cultivation in 100% compost substrates and in soils with admixture of these substrates on the number of fruits obtained during the growing season and the total weight of the fruits was assessed. Fruits were harvested ripe continuously throughout the season, weighed and stored to prepare the resulting mixture for analyses. From the pot experiment in the first year, it appears that tomatoes grown in low-quality EZ soil had the lowest number of fruits (about13 fruits per plant). The yield from ZZ chernozem (about 25 fruits per plant on average) was comparable to the yield of tomatoes growing in a substrate of 100% compost (about 30 fruits per plant on average for all composts used). An 8% admixture of composts to the EZ soil increased the average yield from 13 fruits to 20 fruits per plant. A pot experiment in the second year confirmed the highest average yields from 100% compost substrates, approximately 25 fruits per plant. The yield from EZ was around 15 fruits. Compared to the results from the first-year experiment, the addition of compost substrates to this soil did not significantly increase the yield. Average yields from these mixtures remained at around 15 fruits per plant. In the first year, fruits from plants grown in eroded soil had the lowest total weight (about 300 g per plant on average). In 100% compost substrates, the average fruit weight per plant was 645 g for K-A, 755 g for 1 K-K, and 650 for 2 K-K. The admixture of all types of composts to EZ increased the average mass yields to values of 410 to 495 g, i.e. to the level of quality chernozem ZZ (450 g per plant on average). The fruit weight analysis from the second-year experiment replicated the findings from the fruit number analysis. For 100% compost mixtures, average fruit weights per plant were 700 to 800 g.
Content of selected nutrients and elements in useful parts of crops
Evaluation was done for P, N, K, Na, Ca, and Mg. The element content was measured in dried or lyophilized samples (see above) and determined per kg of dry matter. Subsequently, these values were recalculated using the values of dry matter to fresh matter, both for the biomass of lettuce leaves and for the biomass of fruits from tomato plants. In tomato fruits, a statistically significant difference in content was found for P, Ca, K, Na (both pot experiments of the first and second year) and for N and Mg (pot experiment from the second year). In lettuce leaves, a statistically significant difference in content was found for K and Ca (pot experiment from the first year), but this was not confirmed in the experiment in the following year. In contrast, in this year a statistically significant difference was found for N, P, and Na.
Tab. 1. Average values of heavy metals and arsenic in tomatoes grown in the first-year pot experiment (in mg/kg of fresh matter)
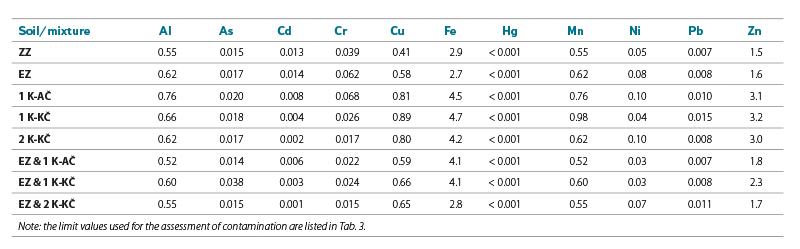
Note: the limit values used for the assessment of contamination are listed in Tab. 3.
The content of nutrients in the leaves from heads of lettuce (Figs. 5 and 7) and also in the tomato fruits (Figs. 6 and 8) was comparable in both pot experiments. The contents of selected nutrients differ between the biomass of heads of lettuce and the biomass of fruits from tomato plants in total numbers mainly because both biomasses have very different dry matter. In the case of lettuce biomass, the dry matter is around an average value of 91 %, in the case of tomato biomass around an average value of 8 % (7 to 11 %). The differences in nutrient content in lettuce and tomato biomass from containers with individual substrates can be seen from the graphs in Figs. 5–8. Due to the conversion to fresh mass, the differences (e.g. in N and K content) are higher in lettuce biomass. The use of substrates from composting resulted in a demonstrably higher content of P, Na and, conversely, a lower content of Ca in tomato fruits in the case of using compost as an admixture. In the pot experiment in the second year, this difference was found only in clean substrates from composting. This was also related to the increase in N content in the biomass (Fig. 8). Similarly, a significantly higher content of all elements with the exception of Ca (a decrease in its content) was found in lettuce biomass from the pot experiment in the second year (Fig. 7).
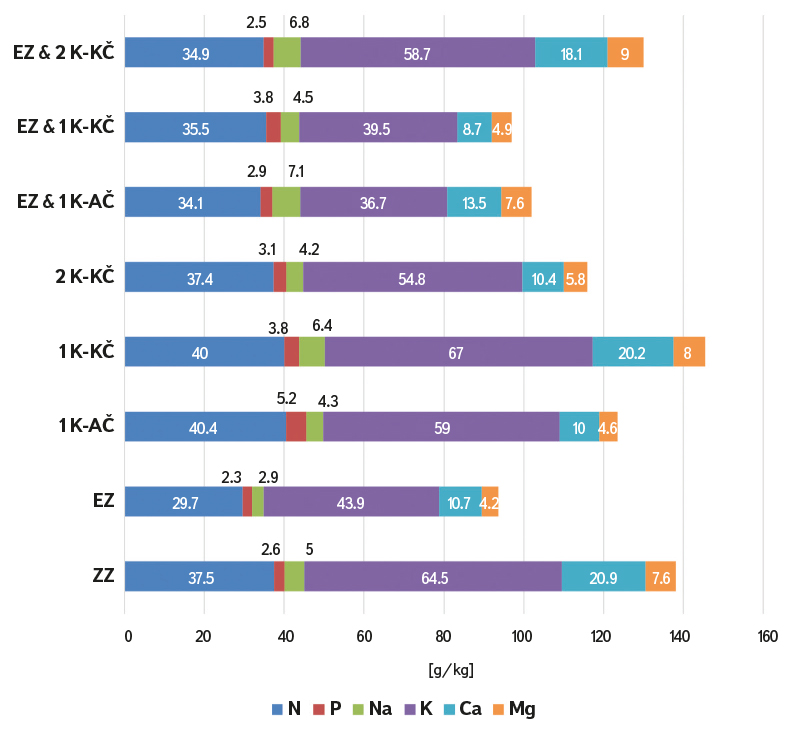
Fig. 5. Average content of nutrients in lettuce heads grown within the first-year pot experiment in g/kg of fresh biomass
Fig. 6. Average content of selected nutrients in tomatoes grown within the first-year pot experiment in g/kg of fresh biomass
Fig. 7. Average content of nutrients in lettuce heads grown within the second-year pot experiment in g/kg of fresh biomass
Fig. 8. Average content of selected nutrients in tomatoes grown within the second-year pot experiment in g/kg of fresh biomass
Content of risk elements in useful parts of crops
The assessment was carried out for the following heavy metals and elements: Al, As, Cd, Cr, Cu, Fe, Hg, Mn, Ni, Pb, and Zn. The analytical procedure for determining the content in biomass and conversion to evaluable results for fresh matter was the same as in the case of the elements listed in the previous sub-chapter.
These results were compared with the limit contents established in the following regulations:
Commission Regulation (EC) No. 1881/2006 (hereinafter referred to as the “Regulation”) setting limits of 0.2 mg/kg Cd, 0.3 mg/kg Pb.
National regulation Decree No. 53/2002 Coll. (hereinafter referred to as the “Decree”), setting limits (in mg/kg of fresh matter): 0.5 As, 0.2 Cd, 0.2 Cr, 10 Cu, 50 Fe, 0.03 Hg, 2.5 Ni, 0.3 Pb, 25 Zn. The Decree was repealed due to accession to the European Community on 1st August 2004. However, it still allows to assess and compare the level of contamination for more metals than the Commission Regulation, where limits are set only for Cd and Pb.
For tomato fruits, no exceedance of the limit values given by the Regulation for either Cd or Pb was detected for individual plants, in both pot experiments. Also, it was not detected that the older, no longer valid limits given by the Decree were exceeded for any monitored element. A comparison of the average values for individual substrate variants (Tab. 1 and 2) shows that no limit contents of risk elements were exceeded either. In tomato fruits, a statistically significant difference in content was found for Zn, Cu, and Cr (both pot experiments), for Ni, Mn, and Cd (pot experiment from the second year), and for Al (pot experiment from the first year).
Tab. 2. Average values of heavy metals and arsenic in tomatoes grown in the second-year pot experiment (in mg/kg of fresh matter)
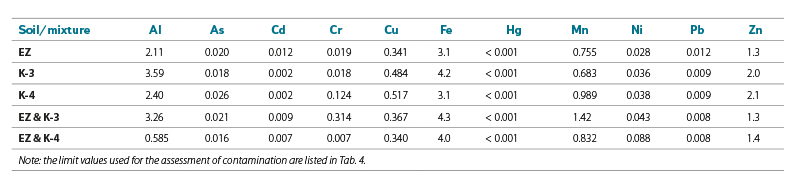
Note: the limit values used for the assessment of contamination are listed in Tab. 4.
Tab. 3. Average values of heavy metals and arsenic in lettuce grown in the first year pot experiment (in mg/kg of fresh matter)
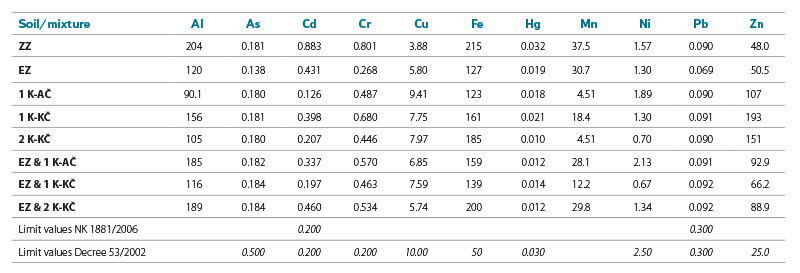
Tab. 4. Average values of heavy metals and arsenic in lettuce grown in the second year pot experiment (in mg/kg of fresh matter)
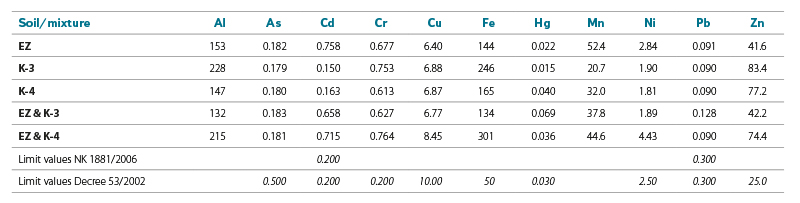
An analysis comparing only container fills consisting entirely of soil or composts determined a statistically significant difference in content for Cd (pot experiment from the second year) and for Al, As, and Zn (pot experiment from the first year). However, in the case of the analysis for the first-year experiment, the analysis was affected by the size of the variance of the two values from the crop pairs, with the mean values lying close to each other.
Limit values were exceeded for lettuce leaves in all leaf samples for Cd from the second-year pot experiment and in all samples from the first-year pot experiment, with the exception of containers filled with K-A compost and K-K compost. The exceedance was valid for samples from all input soils. It was probably caused by the loading of these soils with Cd. When evaluating according to the limits from the Decree, an exceedance was determined in both pot experiments for Zn, Fe, Cr, and from the samples of the second year also in several cases for Ni and Hg. In the case of Hg, these were samples from containers using K-4 compost in mixtures. In the case of Ni, these were two samples out of three from a set of containers with input soil and two samples out of three containers with a mixture of input soil and K-4 compost. In contrast to the experiment from the second year, two samples of the experiment from the first year were determined to exceed the limit value for Cu and Hg. However, it was always only one sample from a pair. It cannot therefore be concluded that some substrate mixtures showed a higher transfer of the given elements to the leaves. In the case of a comparison of average values from individual substrate variants (Tabs. 3 and 4), it follows that the limit contents of Cr and Zn were exceeded in all substrate variants. Ni exceeded the average values for eroded soil and the mixture of eroded soil and K-4 compost from the second-year experiment. Overall, the most problematic was the occurrence of Cd values above the limit. In this case, the limit value in the leaves was already exceeded for the input soils in both experiments. This was also reflected in exceeding the limit value for mixtures of soil and compost. Paradoxically, in the case of 100% compost substrates, the average Cd contents were below the limit in four out of five cases. Therefore, it is not possible to unequivocally prove the negative effect of the application of composted sludge. Of the other risk elements with limits, Pb, Cu, As proved to be unproblematic.
A statistically significant difference in content was found in lettuce leaves for Cd and Mn (both pot experiments), Cu (pot experiment from the first year) and Hg with Zn (pot experiment from the second year). An analysis comparing only container fills consisting of 100 % soil or composts determined a statistically significant difference in content for Zn (both pot experiments) and Cd and Mn (pot experiment from the second year).
The results from the pot experiments can be compared with the results of a number of similar studies and experiments that have been carried out in practically all parts of the world. The aim of these studies and experiments is to verify the possibility of replacing substrates from peat and other non-renewable sources with substrates from composting, including composts used recycle sewage sludge. In the study [22], the authors conducted a pot experiment to investigate the effect of composted sewage sludge (KKOV) applied alone and mixed with chemical fertilizer on the growth and accumulation of heavy metals in lettuce grown on two soils (Xanthi-Udic Ferralosol and Typic Purpli-Udic Cambosol). The experiment included a control (fertilizer containing N, P and K); a composted sludge applied at a rate of 27.54 (KKOV), 82.62 (3KKOV), 165.24 (6KKOV) t/ha; and a mixture of composted sludge and chemical fertilizer (1/2 KKOV + 1/2 NPK). Application doses were determined according to local recommended doses. Application of KKOV increased biomass; content of Cu, Zn, and Pb in lettuce; total metals and metals extracted with DTPA in soil. KKOV at doses of 27.54 and 82.62 t/ha increases plant biomass less than NPK fertilizer alone.
Another study [32] was conducted with the aim of evaluating the potential possibility of using composted sewage sludge (KKOV) as an alternative to expensive peat (PE) for the cultivation of lettuce (Lactuca sativa L.). Five substrates were prepared with different percentages of KKOV and PE in the growth medium. The percentage of KKOV addition to PE was 0 %, 15 %, 30 %, 50 %, and 70 %. The growth media KKOV + PE had very good physical and chemical properties and significant content of plant nutrients, especially P, K, Ca, and Mg. The greatest growth increments and yields were achieved in the growth medium with 30% KKOV and 70% PE from the total volume. Shoot fresh weight, shoot dry weight, root fresh weight, and root dry weight obtained from the growth medium with 30% KKOV and 70% PE were increased by 56.53 %, 43.93 %, 29.46 %, and 67.24 % in comparison with peat substrate. The addition of KKOV as a component of the growth medium increased the concentrations of nutrients (N, P, K, Mg, Ca, Cu, Mn, Zn, and Pb) in the lettuce plant. However, trace element levels in tissues were much lower than phytotoxic levels.
As part of the study [23], a greenhouse experiment was conducted with four lettuce cultivars comparing composted municipal waste with perlite (MSWC + P), composted sludge with perlite (KKOV + P), and peat with perlite (peat + P). Plant biometric parameters measured after 72 days of growth showed that the yield of plants cultivated on KKOV + P was similar to control plants, independent of the cultivar. In contrast, the MSWC + P mixture generally suppressed the formation of biomass, especially in the Murai and Patagonia cultivars. Compared to the peat + P mixture, both compost substrates reduced the accumulation of heavy metals in leaves, with a large effect in the Maximus cultivar. The amounts of Cd and Pb in the edible part were always below the limits set by European regulations.
The authors of the research published in the study [34] prepared a field test in which they grew tomatoes on soil enriched with sludge, soil fertilized with NPK fertilizer, and untreated soil. On soils enriched with the addition of sludge, a higher amount of Cd contained in the above-ground part of tomatoes was found compared to soil with inorganic fertilization. The Cd accumulation in the fruits was low compared to the other analysed plant parts and did not obviously differ depending on the type of soil. The amount of Cd in tomato fruits was an order of magnitude lower than in leaves.
The availability of metals and their accumulation in tomatoes with increasing addition of sludge to the soil was the subject of a study published in the paper of Elloumi et al. [35]. Results showed that soil pH decreased, while salinity, organic C, total N, available P, and reactive forms of Na, Ca, K, and heavy metals increased significantly with increasing sludge application rates. Of the three heavy metals Zn, Cu and Cr, Zn had the greatest ability to transfer from soil to plants. Low translocation of metals from roots to leaves was observed. The use of a dose of 2.5 to 5 % of sewage sludge appeared in the experiment as an effective and cost-effective method for restoring soil fertility.
Zhou et al. [36] found distinct differences in heavy metal concentrations in the edible parts of various vegetables grown in soil contaminated with heavy metals (Pb, Cd, Cu, Zn, and As). Heavy metal concentrations decreased as follows: leafy vegetables > stem vegetables/root vegetables/fruit vegetables > leguminous vegetables/melon vegetables. The ability of leafy vegetables to absorb and accumulate heavy metals was the highest and that of melon vegetables was the lowest.
The mentioned studies will make it possible to assess the risks in the use of substrates from composting sewage sludge from the point of view of the content of risk elements, especially heavy metals, which was also the subject of our experiments. Due to the accumulation of these elements in soils and in biomass during the transfer from sludge to compost, it is necessary to find suitable application doses of substrates that ensure compliance with limit values in soils and biomass, as well as limit the possible risk of phytotoxicity. A study focused on horticultural substrates [29] worked with a dose of composted sludge of only 2 kg of compost with sewage sludge of 2 to 4 kg per 1 m2, which had a positive effect on soil properties and nutrient supply for cultivated vegetables. In our experiments, we verified the benefits and risks of doses of 8 kg of 100% compost per 1 m2, when the risks were below the limit when used for growing tomatoes. In contrast, the use of similar substrates for leafy vegetables appears inappropriate.
In addition to the risks caused by the content of the studied heavy metals and arsenic, it is not possible to ignore the risks associated with the occurrence of other foreign substances and micropollutants in sewage sludge. In the paper [16], our research team presents an overview of drug residues and other micropollutants in sludge before and after composting. It is obvious that, for many of these substances, composting means their reduction or elimination. Styszko et al. [37] monitored changes in the content of selected drugs in sludge during their processing with the aim of safe use. In addition to composting, other methods of processing sludge for substrates usable in agriculture and reclamation are being studied, for example in the form of biochar preparation (e.g. [38, 39]). With the correct dosage, these procedures appear to be promising both from the point of view of eliminating a number of foreign substances (using thermal processes) and from the point of view of enriching the soil with organic matter and nutrients with gradual release.
CONCLUSION
The presented study was focused on checking the possible benefits and risks associated with the use of sludge from domestic and small WWTPs of two main technologies (activation WWTP, reed bed WWTP) within the local circular economy as a source of nutrients for growing selected crops after their processing by composting, which simulated domestic and community small-scale composting conditions. The aim was to verify the possible effects and thus provide information for decision-making in the process of dealing with this sludge. The predominant process is the transfer of sludge to a larger WWTP with sludge management. The study was also carried out with regard to the increasing number of questions about the possibilities of local composting of this sludge and the subsequent use of composts.
Literature reviews of similar studies show that the use of composts to improve soil properties, including composts that include sludge from municipal wastewater treatment, contributes to the support of yields of crops and trees, including various types of vegetables. At appropriate doses, there is no transfer of risk elements to these crops, or only to an extent that complies with the regulations. Both of the presented pot experiments confirmed these assumptions for tomatoes; however, in the case of growing lettuce, the content of some risk elements in the biomass was found to be exceeded. However, this was also influenced by the load on the used soils. The results thus show that local composting with the inclusion of sludge can theoretically achieve quality products that can be used in growing plants, but for selected groups of vegetables it is not suitable (e.g. for leafy vegetables such as lettuce) and excessive contamination of the consumed parts can occur.
The study yielded findings from which it is possible to set appropriate conditions and limits for the use of composts with the addition of sludge from the mentioned groups of WWTPs, considering the content and transfer of risk elements. Microbiological contamination was not monitored as the input analysis of the composts did not show above-limit contamination, or it was zero for most of the composts used. For practical use, however, it would be necessary to conduct a study of the content and transfer of other groups of pollutants, such as drug residues and microplastics.
Acknowledgements
The article was written with the financial support of the project SS02030008 “Centre of Environmental Research: Waste Management, Circular Economy and Environmental Security” and using the results of the project TH02030532 “New procedures for the treatment and stabilization of sewage sludge from small municipal sources” as part of its implementation.
This article was translated on basis of Czech peer-reviewed original by Environmental Translation Ltd.